LIGO scientists have announced the direct detection of gravitational waves, a discovery that won't just open a new window on the cosmos — it'll smash the door wide open.
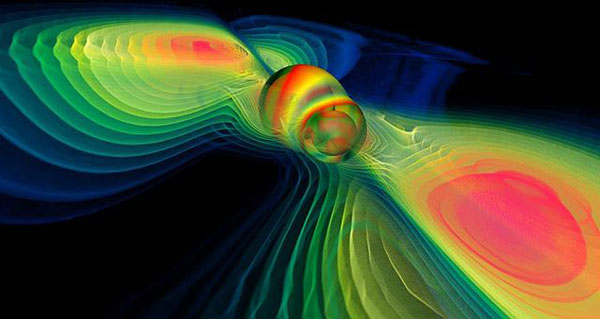
MPI for Gravitational Physics / Werner Benger / ZIB / Louisiana State University
Today, physicists announced the first-ever direct detection of gravitational waves, ripples in the fabric of spacetime predicted by Einstein’s general theory of relativity. Two massive accelerating objects — in this case, a pair of stellar-mass black holes in a death-spiral — passed through spacetime like paddles sweeping through water, creating vibrations that could (barely) be felt on Earth. The results are published in Physical Review Letters.
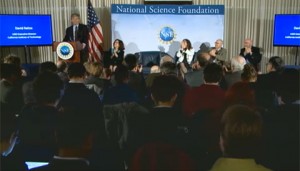
It’s been a recurring theme in history: When scientists open a new window on the universe, they make transformative discoveries. But when LIGO, short for Laser Interferometer Gravitational-Wave Observatory, caught waves from these two colliding black holes, it didn’t just open a new window — it smashed a door wide open, promising a breathtaking new ability to study exotic and otherwise-undetectable cosmic phenomena. Don’t be surprised if LIGO's founders, Kip Thorne, Ronald Drever, and Rainer Weiss, earn free round-trip tickets to Stockholm to collect a Nobel Prize.
Keep up with the latest astronomy and observing news when you subscribe to Sky & Telescope magazine.
The Detection
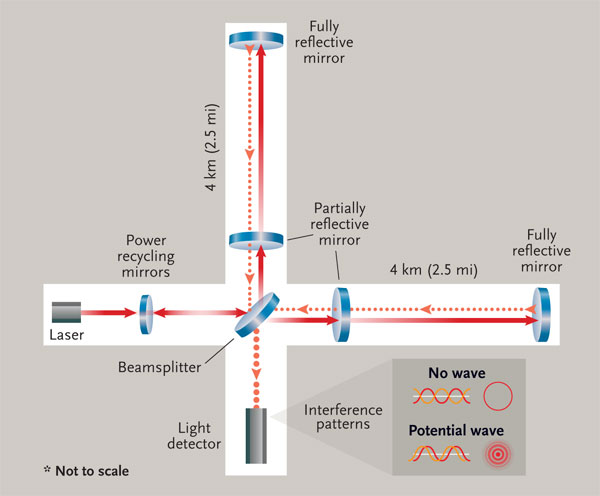
S&T: Leah Tiscione
LIGO consists of two L-shaped facilities, one near Hanford, Washington, and the other near Livingston, Louisiana. At 5:51 a.m. (EDT) on September 14, 2015, both labs caught the gravitational-wave signature of two colliding black holes, shortly after both facilities were turned on following five years of intensive upgrades.
A series of gravitational waves from a distant galaxy first passed through the Livingston detector, then just 7 milliseconds later it passed through the detector in Hanford. Both instruments shoot infrared lasers through 4-kilometer-long arms of near-perfect vacuum. The laser light reflects off ultrapure, superpolished, and seismically isolated quartz mirrors. The passing gravitational waves slightly altered the path lengths in the arms of both detectors by about 1/1,000 the width of a proton. That slight change created a characteristic interference pattern in the laser light, an event LIGO scientists have dubbed GW150914.
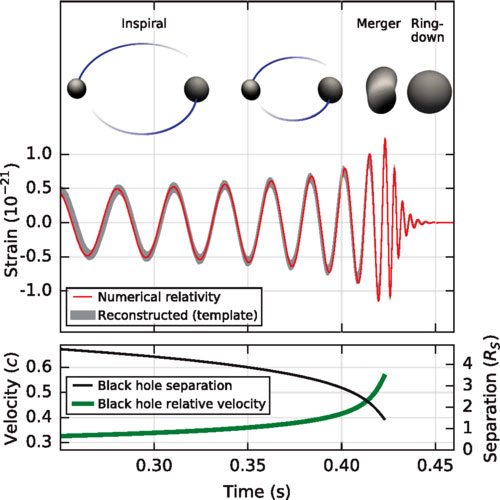
B. P. Abbott & others, "Observation of Gravitational Waves from a Binary Black Hole", Physical Review Letters
Based on the signal’s amplitude (that is, the height of the gravitational wave), team members estimate that the colliding black holes had the masses of about 36 and 29 Suns, respectively. Milliseconds before they merged, these behemoths spun around each other at nearly the speed of light. LIGO watched all three predicted phases of the collision: the black holes’ death spiral and ensuring merger, as well as the ringing of the merged object as it settled into its new form.
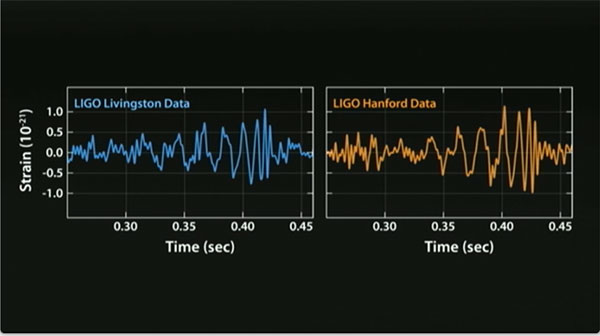
LIGO
The merged black hole contains about 62 solar masses, so it's short three solar masses — the gravitational waves themselves carried away three solar masses worth of energy.
The minuscule difference in the waves’ arrival times at the two facilities was exactly what’s expected for gravitational waves, which travel at the speed of light. The LIGO team claims a 5.1-sigma detection, meaning the odds of the signal occurring by chance are about one in 3.5 million.
With only two detectors, LIGO can’t pinpoint the source’s exact location or host galaxy — it could come from anywhere within about 600 square degrees of sky, somewhere near the Large Magellanic Cloud in the Southern Hemisphere sky. Nor can they exactly pinpoint its distance, but measurements show the source lies between 700 million and 1.6 billion light-years away.
The beginning of this video (at 0:07) shows an all-too-brief simulation of the merging black holes and the extreme warping of spacetime around them:
A New Window on the Cosmos
The direct detection of gravitational waves opens up an entirely new spectrum that doesn’t involve any form of light. “It’s a spectrum that carries entirely new kinds of information that have so far been largely invisible,” says physicist Robert Owen (Oberlin College).
Or, as Eric Katsavounidis (MIT and LIGO team member) puts it, "This is the end of the silent-movie era in astronomy."
Previously, radio astronomers studying pairs of neutron stars, the crushed, spinning remains of massive stars, had revealed compelling indirect evidence of gravitational waves. Einstein’s general theory of relativity says that gravitational waves should carry away orbital energy, and indeed, these pulsars’ orbits spiral inward at exactly the rate relativity predicts. Joseph Taylor and Russell Hulse shared the 1993 Nobel Prize in Physics for discovering the first of these systems.
But direct detection has remained elusive because of the incredible difficulty of catching gravitational waves. Merging binaries involving black holes or neutron stars generate stupendous amounts of energy. “In terms of gravitational waves, for that one millisecond prior to merger, this binary black hole system was ‘brighter’ than all the rest of the universe combined!” Owen says. In fact, later calculations say that at its peak, the merging black was putting out 50 times more energy than the rest of the universe.
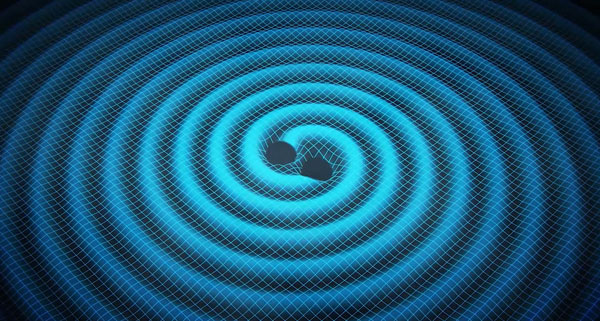
Swinburne Astronomy Productions
But the waves are incredibly difficult to detect because gravity is the weakest of the four known forces of nature, the strength of the waves fall off sharply as they traverse space, and because matter barely feels the presence of gravitational waves. “The gravitational waves from a distant galaxy that are detectable to LIGO are squeezing and stretching the Milky Way Galaxy by the width of your thumb,” says LIGO science team member Chad Hanna (Penn State University).
The National Science Foundation-funded $500 million LIGO experiment has been on the lookout for gravitational waves since 2002. But only recently, after a five-year rebuild and redesign to improve LIGO’s sensitivity, did the facilities have a realistic chance of catching these subtle spacetime ripples. LIGO began its first “advanced” observing run last fall, but improvements continue and future runs will have at least twice the sensitivity and enable LIGO to survey ten times the volume of space.
Theorists predict Advanced LIGO should catch an additional five binary black hole mergers in its next observing run. They also expect roughly 40 binary neutron star mergers every year it runs, and an unknown number of signals from black hole-neutron star mergers and supernovae. It's even possible that LIGO could detect exotic cosmic strings.
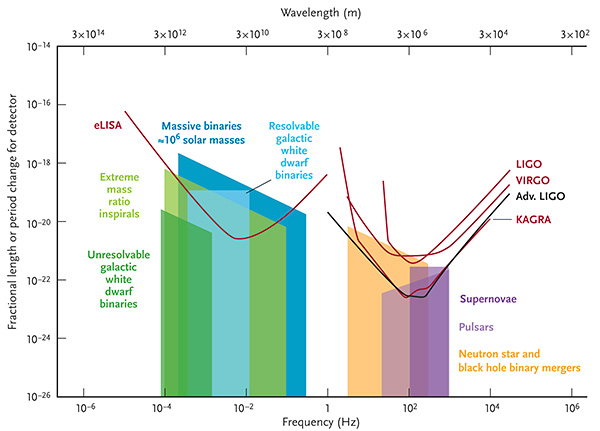
S&T: Leah Tiscione; Source: C. J. Moore et al. / arXiv.org 2014
The direct detection of gravitational waves represents another triumph for Einstein, almost exactly 100 years after he predicted their existence — and despite the fact that he never thought they’d be detected. But as LIGO builds up a catalog of events in the coming years, and as other advanced detectors come online in Europe and Japan, physicists will be scrutinizing the waveforms in detail to see how closely they conform to general relativity’s predictions.
Though this black hole merger went entirely according to Einstein’s predictions, scientists hope to eventually see discrepancies that could provide vital clues to new physics, potentially reconciling contradictions between relativity and quantum theory.
“Gravitational-wave measurements will allow us to directly probe some of the most violent events in the universe, to directly measure the most tumultuous dynamics of spacetime geometry,” says Owen. “Gravitational waves would allow us to probe how spacetime really behaves under the most radical of circumstances.”
LIGO will prove a gold mine for astronomers: enabling them to study and build up a census of neutron stars, stellar-mass black holes, and other dim or otherwise impossible-to-detect objects in faraway galaxies. And LIGO also offers the tantalizing prospect of discovering new types of objects and phenomena hitherto unknown to science.
“We want to give ourselves plenty of opportunity to be surprised,” says Hanna. “We don’t want to open a new window to the universe and then refuse to look outside because we think we know what we’ll see. We expect the bread-and-butter sources, but we certainly hope it doesn’t stop there.”
Further Resources
- Get the prescient cover story of Sky & Telescope's December 2015 issue.
- LIGO scientists get their feet wet to explain the historic detection:
- What are gravitational waves? Read a primer from UniverseToday.com on the phenomenon.
- How does LIGO detect gravitational waves? Piled Higher and Deeper (PhD Comics) explains:
- What upgrades made Advanced LIGO's detection possible? Read our story on ALIGO's first first observing run last September.
- Want more? Check out an award-winning 20-minute documentary on LIGO, as well as a slew of videos describing how the European space-based gravitational-wave observatory, eLISA, will work, and more in our Beyond the Printed Page resources.
- Take part in the science with Einstein@Home. Sign up and enlist your computer's idle time to help analyze gravitational-wave data.
Comments
Donald Bruns
February 11, 2016 at 12:19 pm
Congratulations to the LIGO teams! A former colleague of mine joined LIGO about 1994, so he must be very happy now after working 22 years on this incredibly difficult measurement. As part of the Einstein General Relativity centennial, I also hope to contribute by re-measuring Einstein's prediction of the sun's deflection of starlight, visible during total eclipses. This hasn't been done since 1973, but I'll be doing the experiment in 2017 using modern technology.
You must be logged in to post a comment.
Mark Looper
February 11, 2016 at 2:29 pm
And best of luck to you in replicating the starlight-deflection experiment next year! It's been a long time since I studied GR; do I remember correctly that the prediction for deflection is exactly twice what would naively be predicted from Newtonian gravitational mechanics? That is, observing deflection by itself proves nothing; the test is whether the additional effects of spacetime curvature beyond Newtonian physics are quantitatively measured. And, while I'm asking -- do you know of any online educational resources, like the fine selection linked to by the article above, that discuss the starlight-deflection measurement? Thanks!
You must be logged in to post a comment.
Donald Bruns
February 11, 2016 at 5:03 pm
You might want to start with the Wikipedia article on "Tests of general relativity", and follow those links. Depending on what level of information you are looking for, there are plenty of web pages, books, and articles to choose from. I know that repeating this deflection experiment is very tough, the most difficult one I've ever attempted. I hope to measure the correct value to within 1%, or five times better than those done last century with glass negatives!
You must be logged in to post a comment.
randallpaul
January 23, 2017 at 1:06 pm
Will primordial gravitational waves also be detectable, furthering the support of Alan Guth's inflation theory?
You must be logged in to post a comment.
Anthony Barreiro
February 11, 2016 at 2:27 pm
Unlike the BICEP2 team's hasty 2014 press conference announcement that they had detected gravitational waves from cosmic inflation (we later learned that they had more likely found dust in the milky way), the LIGO team didn't officially announce their discovery until they were sure of a 5-sigma detection and had gotten a paper published in a peer-reviewed journal. This is how science is supposed to work. Congratulations! GW150914 will go down in history. I just wish they had given it a catchier name.
You must be logged in to post a comment.
Mark Looper
February 11, 2016 at 2:29 pm
"The Horrendous Space Kablooie!"
You must be logged in to post a comment.
Frank-ReedNavigation.com
February 11, 2016 at 6:07 pm
You wrote: "GW150914 will go down in history. I just wish they had given it a catchier name."
How about "Gravitational Wave 2015 September 14"?
Oh, wait.
😉
You must be logged in to post a comment.
Tom Hoffelder
February 12, 2016 at 9:03 am
So wonderful to see science working the way it should!!! If only it happened more often. Thanks for pointing out the differences!
You must be logged in to post a comment.
Frank-ReedNavigation.com
February 11, 2016 at 6:12 pm
Well, finally: an Internet rumor turns out to be ONE HUNDRED PERCENT TRUE! Congratulations to Lawrence Krauss for putting his credibility on the line and breaking this story in a Twitter post on September 25, 2015, just ELEVEN DAYS after the detection.
You must be logged in to post a comment.
February 13, 2016 at 11:35 am
Larry shot his credibility to me by jumping the gun to announce OTHERS work.
I would not have such an indiscreet person him on any research team of mine, either.
You must be logged in to post a comment.
Frank-ReedNavigation.com
February 11, 2016 at 6:19 pm
Although the story has been an open secret for five months, I think it's great that they waited for the official announcement synchronized with the published paper. They double-checked. They triple-checked. And they quadrupole-checked. HA!
It's probably a very old pun among gravitational wave specialists... 😀
https://en.wikipedia.org/wiki/Quadrupole#Gravitational_quadrupole
You must be logged in to post a comment.
Fred Shuman
February 11, 2016 at 7:13 pm
Yeah, it probably is, but it's a good one nonetheless.
You must be logged in to post a comment.
Fred Shuman
February 11, 2016 at 7:21 pm
This is absolutely incredible news!!
I would like to point out that this isn't the first non-EM astronomical detection/observation — don't forget SN1987A, seen by Kamiokande-II (and others?); as well as Homestake and Ray Davis' lifetime supply of cleaning fluid — we've seen some things by way of neutrinos, after all!
But this really is a tremendous leap forward!!
And just the first of a constellation of grav-wave detectors. Exciting times are afoot!
You must be logged in to post a comment.
Frank-ReedNavigation.com
February 11, 2016 at 10:43 pm
Yes, I had trouble with that distinction myself earlier today. Setting aside neutrinos, what about more ordinary "cosmic rays" as another example of "radiation" off the EM spectrum? I decided that, if we want to be careful with our terminology, then we have to talk about "massless boson" radiation. With that careful splitting of hairs, there's only the electromagnetic spectrum and the gravitational radiation spectrum. And in fact, with that condition, they're the only possibilities in the Standard Model. There's astronomy by photons, and there's astronomy by gravitons. The rest is just a bunch of messy fermions. 😉
You must be logged in to post a comment.
Julio-Vannini
February 12, 2016 at 8:31 am
This is terrific news! Sailing the waves of gravity and light! Woohoo!
You must be logged in to post a comment.
Mick-Snyder
February 12, 2016 at 10:53 am
Hmm. All very good news that LIGO has seen gravitational waves and a triumph for amazing precision engineering, coupled with excellent process to avoid another BICEP2 issue. And they say that Einstein's General Relativity equations agree exactly with observations.
But hang on. That is so IF the two black holes were 36 and 29 suns AND the distance was between 700 million and 1.6 billion light years AND the remaining mass is 62 suns THEN that is what GR would predict.
But we don't know what the masses actually were or are, or the true distance. So it seems to me that they have certainly seen an GW event but the hype over its "proving" GR to great exactitude is a bit less certain. The measurements of orbtal decay that have been made in the last few years seem to be a better "proof" of GR.
The real news is that we have now got technology in place with working detectors which we can use for ongoing research into GW.
You must be logged in to post a comment.
February 12, 2016 at 2:55 pm
I'm just a layperson with a calculator, but the article says LIGO Hanford received the identical waveform 7 milliseconds after LIGO Livingston. Google map says the two locations are approx 1875 miles apart.
1875 mi divided by 186,000 mi/sec = .01 seconds or 100 milliseconds. Why the difference?
You must be logged in to post a comment.
Monica Young
February 12, 2016 at 3:36 pm
An excellent question, and it demonstrates how the scientists were able to get information on the source's location on the sky. You're assuming that the wavefront hit Livingston first, perpendicularly, and then traveled onward to Hanford. But the wavefront wasn't perpendicular. It hit Livingston at an angle, so part of the wavefront was already well on its way to Hanford. In other words, the difference in arrival time tells you the angle of the wavefront, and that's what enabled scientists to narrow down its location on the sky somewhat. (If they had had a third interferometer running, they would have been able to localize the source much better.)
You must be logged in to post a comment.
SK
February 12, 2016 at 6:39 pm
Thanks, Monica! A clear and helpful answer! Does this mean that we know the location of the event to within a cone of a specified angle?
You must be logged in to post a comment.
Anthony Barreiro
February 12, 2016 at 8:56 pm
That sounds like a great reason to build a third interferometer. Are there any proposals or plans?
You must be logged in to post a comment.
February 12, 2016 at 10:48 pm
There are European based interferometers (Germany and Italy) and one in in Japan. I believe they're all undergoing upgrades to increase their sensitivity. Another proposal is for an Australian observatory. The LIGO report gives an area (I think with 90% confidence) of 600 square degrees for the source location. A worldwide interferometer collaboration would give much improved positional information for future GW work, and I guess it might enable radio and optical telescopes to partake in observation of gravitaional wave sources.
Then there's the NASA proposal for a space based interferometer, LISA which would have a triangular configuration and baseline of 5 million kilometres for each arm!
You must be logged in to post a comment.
Anthony Barreiro
February 16, 2016 at 4:09 pm
Thanks everyone for your information about current and planned gravitational wave detectors. One small correction to Dr. Strange's post: eLISA is a project of the European Space Agency, not NASA.
You must be logged in to post a comment.
February 13, 2016 at 11:10 am
I understand there are discussions of additional detectors in Italy and India.
You must be logged in to post a comment.
February 15, 2016 at 7:49 pm
There is a third interferometer located in Italy (Virgo) which employs 3 kilometre arms and is in collaboration with LIGO. It is undergoing upgrades to increase its sensitivity (advanced Virgo),
which are due for completion this year. There are others in Germany (GEO600) and in Japan, although they don't have the sensitivity of LIGO. A space borne interferometer (LISA) is planned, but construction is not expected until the early 2030's.
You must be logged in to post a comment.
February 12, 2016 at 10:07 pm
Another consideration is that the 3,000 km separation between Hanford and Livingstion is (presumably)
the measured 'surface' distance. The gravitational waves would take a shorter straight line path 'through' the earth, resulting in a shorter travel time between the two sites.
You must be logged in to post a comment.
February 13, 2016 at 12:59 pm
Thanks for that. I was thinking in 2 dimensions...not good for an astronomer. The 2 stations could have received a valid signal even simultaneously. 2nd layperson question: Since the spinning stars are in hyper-gravitational environment, why don't they break apart before impact? Are neutron stars that tough?
You must be logged in to post a comment.
Mike-Jewell
February 13, 2016 at 1:25 pm
Actually, that's 10 milliseconds, not 100.
And as Ms Young points out that would be the delay if the source was perfectly lined up with the detectors' baseline. So, the offset angle (about 45 degrees?) seems to me to produce a big ring shaped area of the sky for the source location.
Is that right or is there some other characteristic of the signal that can further pin-point the location?
You must be logged in to post a comment.
February 13, 2016 at 10:32 pm
Since gravity waves would travel in a straight line between the Hanford and Livingston sites, I believe this is the distance (rather than the 3,000 km surface measurement) that needs to be used when accounting for delay between the interferometers. The shorter delay path would give a shallower offset angle.
You must be logged in to post a comment.
Frank-ReedNavigation.com
February 19, 2016 at 4:31 am
The difference between the (great circle) surface distance and the straight line distance through the body of the Earth is only about 1% for these locations. That's insignificant.
You must be logged in to post a comment.
February 12, 2016 at 8:10 pm
Yes to Monica's explanation, but note also (Mr. Layperson ) that 0.01 is ten milliseconds, not a hundred milliseconds. This is closer to what would be expected. But the advancing wavefront should take at MOST the light travel time from Livingston to Hanford and then only if it was propagating in a line colinear with the two stations. If the wavefront hit the earth "perpendicularly" ) relative to the two LIGOs, it would have been simlutaneous. Since the actual time interval was 7 ms, the wavefront made about a 45 degree angle (arcsin 0.7) with the line connecting the two stations.
You must be logged in to post a comment.
February 13, 2016 at 11:18 am
What would be the effect on a 'nearby' observer (say a few light years away from the event, a billion times closer than us)? I guess they would experience gravitational waves a billion billion times stronger than on Earth (assuming an inverse square law), resulting in compression and expansion of one part per thousand, meaning the surface of an earth-sized planet would move by a few km. But would this be a catastrophic event or would few people notice because everything (on the hypothetical planet) would be affected simultaneously?
You must be logged in to post a comment.
Frank-ReedNavigation.com
February 13, 2016 at 4:21 pm
Quentin, Although "everything on the hypothetical planet would be affected simultaneously" and with exactly the same gravitational acceleration, it's the other forces that would lead to catastrophe. For a textbook example, imagine two bowling balls welded to the ends of a 10 meter steel bar and nearby two bowling balls affixed to the ends of a spring that's also 10 meters in length. When these two systems are struck by gravitational radiation in the right direction, the bowling balls on the ends of the spring will oscillate back and forth nearly in sync with the incident radiation and with a large amplitude. Meanwhile the balls on the ends of the steel bar will be held in place by the greater internal forces in the steel. They will barely move.
This is all a lot easier to understand if we remember that gravitational fields are frequently best understood in terms of tidal forces. The expression "space-time curvature" sounds all "timey-whimey" (to borrow a Time Lord expression) and incomprehensible. But, in fact, we're talking about tides, and we can compare with ocean tides.
The Moon generates a weak, very slowly changing tidal field here on the Earth raising high tides in the oceans (in an idealized model) on the near and opposite side and drawing low tides in between. Although those tidal fields affect all matter with the same acceleration, different types of matter react differently. The Earth's rocky crust itself moves up and down a few cm in response to the Moon's tides in a relatively simple direct relationship to the driving force, while the oceans respond generally with motions about 50-100 times greater in magnitude and in a highly complex pattern of resonant and semi-resonant oscillations. Rock moves a little. Water moves a lot. These different motions occurring under the same tidal gravitational field.
Gravitational radiation can be treated as a rapidly varying tidal field that is in motion at the speed of light. This can be justified mathematically (it's not an analogy). And when a strong gravitational wave strikes a planet, some parts will move easily, others will be constrained by internal forces. High tides will be raised in one area, and then a fraction of a second later, they will be replaced by low tides (note that with oceans, the water will not have time to flow, so this doesn't mean that the resulting motions would be just like tides here on Earth). With the right strength and frequency, yes, it would be catastrophic. You could shake a planet apart ...or at least the parts of it that we are "attached" to ...in both senses of the word.
Frank Reed
ReedNavigation.com
Conanicut Island USA
You must be logged in to post a comment.
February 14, 2016 at 4:23 am
Thanks Frank, the clarity of your reply has helped to clarify my own thinking. I was wrong to use the word 'simultaneously' in describing the effects of a gravity wave on a planetary sized object. The point on the surface nearest to the event would experience a wave front a few tens of milliseconds before the point on the opposite side, and the wavelength just before the merger (when the amplitude would be greatest) was around 1,200 km (corresponding to a frequency of 250 Hz). So plenty of scope for differential effects, as you say.
There's an idea here for a nice sci-fi short story. Two nearby, quiescent black holes approaching merger would be nearly undetectable for millennia before an abrupt catastrophic merger. We now know there's no such threat to us, because it would have shown up clearly even on the first generation of Ligo. That's a relief!
You must be logged in to post a comment.
Robert-Casey
February 13, 2016 at 5:46 pm
The event happened on my father's 100th birthday. 🙂 Yes, I realize that this is only significant to my family, but wanted to mention it. 🙂
Now that we can directly detect gravitational radiation, I wonder if it could be used for communications, like radio waves. But it took 3 solar masses worth of energy to be barely precepeable here, albeit a billion light years away. It will be a while before that technology is developed.
You must be logged in to post a comment.
February 15, 2016 at 7:20 pm
Since GW150914 is located in the Southern Celestial Hemisphere (in the vicinity of the LMC), does this mean that the gravitational waves traversed the Earth before interception by LIGO? Is that even possible, or does the interferometer require a view of the sky for GW detection?
You must be logged in to post a comment.
Frank-ReedNavigation.com
February 16, 2016 at 5:33 pm
Yes, gravitational radiation will pass right through the Earth without measurable distortion. Gravitational waves are fundamentally 'waves of tidal force'. And just as the Moon's tidal force has no problem raising tides on the opposite side of the Earth --its tidal force passing right through the Earth-- so also gravitational radiation from extremely distant astronomical sources passes straight through the Earth. There is some small distortion of the wavefronts as they pass through the Earth, eventually leading to gravitational lensing of gravitational radiation, but since significant sources of gravitational radiation are presumably so rare, the probability of observing any measurable lensing would be quite low.
You must be logged in to post a comment.
February 16, 2016 at 10:41 pm
Thanks for your response.
Another question if I may : The distance between the Hanford and Livingston sites has been given as 3,000 km or so. I assume that this spacing has been chosen to give a 'straight line' delay of exactly 10 milliseconds for GWs, in which case the quoted distance refers to a chord through the earth, rather than the 'surface' distance between the interferometers (which would be somewhat larger due to its curvature). The factor of 0.69 appearing in the measured delay of 6.9 ms would then give an indication of the wavefront angle.
You must be logged in to post a comment.
Frank-ReedNavigation.com
February 19, 2016 at 4:38 am
For the locations of the two LIGO detectors, the chord distance through the body of the Earth compared to the distance across the surface of the Earth (great circle distance) is only about 1% shorter. This is not significant for the analysis at yet. In a few years, yes, with more detectors active, but it doesn't affect the numbers at the current level of analysis.
You must be logged in to post a comment.
February 19, 2016 at 11:51 pm
Yes, I see what you mean, given the larger uncertainty in delay time (+0.5ms, -0.4ms)
between the Hanford and Livingston sites.
As you say, the addition of more detectors will give more useful information wrt position. The figure of 590 square degrees given for GW150914 is a large search area, and the combination of LIGO with an upgraded Italian Virgo interferometer is sure to improve angular resolution to an extent that would allow follow-up observations at optical and other wavelengths.
You must be logged in to post a comment.
You must be logged in to post a comment.