Scientists with the gravitational-wave observatory announce another discovery, this time of a black hole merger twice as far away as previous detections.
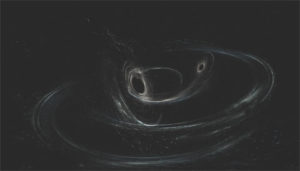
LIGO / Caltech / MIT / Sonoma State (Aurore Simonnet)
What was once a pipe dream is becoming old hat. Scientists with the Laser Interferometer Gravitational-Wave Observatory (LIGO) have announced their discovery of another black hole merger, revealed when the gravitational waves that were created by the cosmic consolidation changed the length of the two LIGO sites’ arms by approximately one-thousandth the width of a proton.
Gravitational waves are ripples in the fabric of spacetime, created by accelerating masses. Along with black holes, gravitational lenses, and other fun phenomena, they’re a prediction of Einstein’s masterwork vision of gravity, the general theory of relativity.
The newly announced event, called GW170104, squeezed and stretched LIGO’s 4-km-long arms on January 4th, during the observatory’s (ongoing) second observing run. The larger of the two black holes had a mass between 25 and 40 times that of the Sun; the smaller, between 13 and 25 Suns. The resulting black hole has a mass of about 50 solar masses, with a couple of solar masses carried away as gravitational radiation, the collaboration reports June 1st in Physical Review Letters.
Based on how “loud” the signal was, the researchers conclude that the merger happened roughly 3 billion light-years away, somewhere in a long, skinny region on the sky spanning roughly 1200 square degrees.
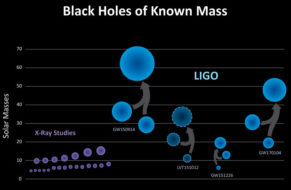
LIGO / Caltech / Sonoma State (Aurore Simonnet)
This is the third firm detection of gravitational waves. LIGO scientists detected the previous two events in September and December 2015, announcing them each in 2016. (A third iffy detection, in October 2015, was not strong enough to rise above “candidate” status.) These events all appear to come from the final moments of the merger of two black holes, each object having about ten or more solar masses.
Before LIGO, astronomers could only “see” this type of black hole when it siphoned gas from a companion star, heating the gas up to X-ray-emitting temperatures. The objects LIGO first detected, with masses of more than 20 Suns, came as a surprise — many theorists didn’t think such beefy stellar-mass black holes existed. (Supermassive black holes, the ones skulking in galaxies’ cores, are a distinct population: Unlike the LIGO objects, they haven’t reached their current sizes by forming from a single star.)
The final mass of the black hole produced by the latest merger lies betwixt those of the previous two LIGO discoveries. It also happened approximately twice as far away as those events.
GW170104 is the first discovery from LIGO’s second observing run, at least that we know of. The team has circumspectly noted that they’ve identified seven “triggers,” but deputy spokesperson Laura Cadonati (Georgia Tech) cautions that she and her colleagues cast a wide net when looking for potential gravitational waves, so many triggers may not pan out.
Deciphering the Gravitational-Wave Wiggle
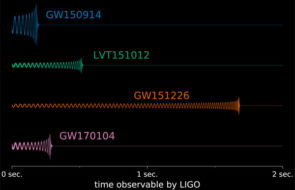
LIGO / Caltech / MIT / University of Chicago (Ben Farr)
Scientists derive things like the black holes’ masses, spin, and distance based on a careful breakdown of the gravitational wave signal. The frequency of the gravitational waves, for example, is inversely proportional to the total mass of the black holes: Higher frequency means lower mass.
One aspect of GW170104’s signal has astrophysicists excited: the black holes’ spins. Although the team can’t determine the exact direction and speed of the two black holes’ spins before the merger, the gravitational wave pattern does indicate that at least one of the black holes was spinning in the opposite direction from the one in which the two objects were orbiting each other.
The reasoning goes something like this: If the two black holes were spinning exactly aligned with the axis of their orbit, they would have needed to shed some of the system’s total rotational energy before they could merge. Such a merger would take a few more orbits than if the spins weren’t aligned, Cadonati explains. But the team didn’t see this “hang-up” effect, so they think things weren’t perfectly lined up.
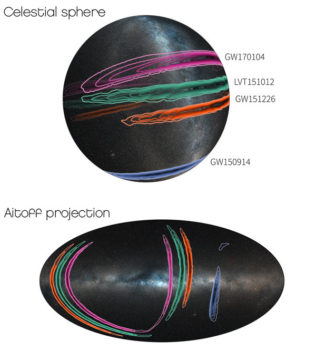
Contours: LIGO / Caltech / MIT / Leo Singer, Milky Way Image: Axel Mellinger
This hint of a misalignment is tantalizing. If the two black holes formed from two stars that began life together as a binary system, then it’s more likely that they would be spinning in roughly the same way. But if the black holes joined up after they formed — say, by sinking to the center of a dense stellar cluster — then their two spins could easily be totally different. “This is an important clue in understanding how black holes form,” Cadonati says.
The spin values themselves might provide another clue. All three of the final black holes whose birth LIGO has detected spin at rates that are about 70% of the maximum allowed. This result matches recent calculations by Maya Fishbach (University of Chicago) and others, suggesting that when black holes of similar masses merge, the resulting object will have a spin rate around this value. If this correlation holds up, then whenever we find a black hole with this spin, we might be able to say that it’s the product of a merger.
Part of the great allure in studying black holes is that, as gravitational objects, they’re perfect laboratories for testing our understanding of gravity. Thus far, all the gravitational-wave events detected are cookie-cutter examples of what scientists expected to see, based on Einstein’s general theory of relativity. Despite murmurs of exotic echoes in the first event’s gravitational wave signal, the LIGO team is skeptical of that analysis. As of yet, scientists have found no clear deviation from Einstein’s gravity.
But what they have found is just as cool: excellent evidence that black holes are real.
References:
B. P. Abbott et al. “GW170104: Observation of a 50-Solar-Mass Binary Black Hole Coalescence at Redshift 0.2.” Physical Review Letters. June 2, 2017. (Published June 1st.)
Maya Fishbach, Daniel E. Holz, Ben Farr. “Are LIGO's Black Holes Made from Smaller Black Holes?” Astrophysical Journal Letters. May 10, 2017.
If you’d like to know more, read the behind-the-scenes story of the first gravitational-wave detection and what we’ve learned from these spacetime ripples in our September issue, on newsstands August 1st.
Comments
Lindsay
June 2, 2017 at 6:40 pm
Very interesting Camille!
In the chart comparing waveforms, I assume the abrupt stop is when the two black holes merge into one?
Is what you refer to as 'loudness' proportional to the amplitude of each waveform shown in the chart?
Why does the amplitude increase just before the signal stops?
You must be logged in to post a comment.
Graham-Wolf
June 2, 2017 at 8:44 pm
Well done LIGO. Keep it up!
Worth every single dollar spent on it.
Graham W. Wolf
46 South, NZ
You must be logged in to post a comment.
You must be logged in to post a comment.